Study Demonstrates Ultra-Low-Power Second-Order Nonlinear Optics on a Chip
By Taha KhanAug 9 2022 Reviewed
by Laura Thomson
In an article published in Nature Communications, researchers have demonstrated efficient frequency doubling and parametric oscillation with a threshold of tens of micro-watts in an integrated thin-film lithium niobate photonic circuit.
Study: Ultra-low-power second-order nonlinear optics on a chip. Image Credit: nevodka/Shutterstock.com
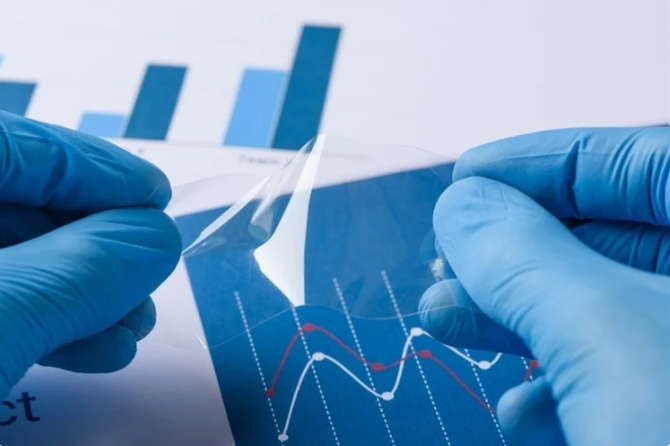
The evolution of silicon photonics gave rise to the development of high-performance, complex optical systems for sensing, communication and quantum and classical information processing. Many applications would considerably benefit from including adaptable nonlinearities in addition to linear passives, modulators, and detectors.
Amorphous silicon nitride is used to make integrated silicon-based photonic circuits that restrain light in dispersion engineered waveguides and resonators, enhancing the third-order optical nonlinearity. These have demonstrated squeezed light generation, wavelength conversion and frequency combs.
Researchers have put continuous efforts into incorporating second-order nonlinearity to improve the tailorability and efficiency of such devices, enabling stronger interactions at lower power and reducing competing nonlinear processes that emerge.
Heterogeneously integrating a non-centrosymmetric material or breaking a crystal's symmetry can introduce second-order nonlinearity.
Similarly, χ(2) nonlinear materials such as lithium niobate (LN) or aluminum nitride can be used to build photonic circuits. Lithium niobate can be periodically poled to compensate for phase mismatch caused by dispersion supporting Kerr nonlinearity, a large electro-optic coefficient, and high-Q optical resonances.
Demonstrating Ultra-Efficient Resonant χ(2) Nonlinear Optical Functions on a Chip
The researchers demonstrated ultra-efficient resonant χ(2) nonlinear optical functions on a chip
incorporating quasi-phase-matching with a nonlinear optical resonator. They overcame the parasitic effects that limit the performance and stability of integrated lithium niobate devices, demonstrating second-order processes such as an optical parametric oscillator (OPO) that previously could only be observed in lithium niobate bulk resonators.
The researchers operated OPO at ambient temperature over degenerate and nondegenerate regimes and demonstrated tuning of the emission spectrum over 1 THz by modulating the pump's frequency over hundreds of MHz.
They also demonstrated frequency doubling, which results in greatly improved effective third-order nonlinearity and, ultimately, cascaded parametric oscillation. The coupled-mode theory that has been developed in this study provides an accurate description of the dynamics and validates the operating modes of the device.
A thin film of X-cut LN was used to make waveguides, having χ(2) tensor and the largest electro-optic components parallel to the chip surface. Recent demonstrations of cryogenic frequency, frequency combs, telecommunications modulators and sources that exhibit quantum correlations have all used this orientation. Magnesium oxide (MgO) doped LN was used to inhibit pump-induced absorption and reduce the photorefractive damage generally incurred by devices constructed using undoped congruently grown LN.
Fabrication of the Devices
All devices were fabricated using X-cut thin-film lithium niobate-on-insulator (LNOI) wafers. A lithium niobate of 500 nm bonded with a silicon dioxide layer of 2 μm thickness on top of a lithium niobate handle wafer constitutes the material.
Electron beam lithography was used for patterning the optical devices, whereas Argon ion milling with accelerating voltage of 750 V and 15 sccm AR flow was used to transfer it. The width of the waveguide was set to 1.2 μm and etch depth of 300 nm, leaving a 200 nm slab of lithium niobate underneath the waveguide. PECVD silicon dioxide of 700 nm thickness was deposited as cladding at 350 °C.
Before waveguide fabrication, periodic poling was performed using electron-beam evaporated Cr electrodes to invert the crystal domains. However, the researchers poled only one side of the racetrack resonator, and electrodes were later removed after the completion of the poling step.
The bandwidth of the quasi-phase-matching was approximately 10 nm due to the waveguide's normal dispersion. A DISCO DFL7340 laser saw was used to prepare the chip edge facet. A periodic array of damaged locations acting as nucleation sites for crack growth was created by focusing high-energy pulses into the substrate resulting in a smooth and uniform cleave.
Significant Findings of the Study
Quantum entanglement is produced as light changes from one wavelength to another via second-order nonlinear optical processes. Developing chip-scale devices to manage these interactions substantially expands the scope of photonics effectively. The current photonic circuits use third-order optical nonlinearity; however, developing a similar platform for second-order nonlinear optics is still challenging.
This study shows effective frequency doubling and parametric oscillation in an integrated thin-film LN photonic circuit with a threshold of tens of micro-watts. Furthermore, by changing the pump frequency by hundreds of megahertz, the researchers could operate the parametric oscillator in degenerate and nondegenerate modes at room temperature and tailor its emission across the one terahertz range. Finally, parametric oscillation caused by cascaded second-order processes was shown.
Future Prospects
The ultra-efficient second-order nonlinear photonic circuits such as parametric oscillators and frequency doublers demonstrated in this research are expected to be advantageous for various new low-power and quantum applications in the near future. High-performance devices and components being created for the thin-film lithium niobate platform are expected to soon realize a novel class of versatile integrated photonic technologies.
Integrated ultra-low-power OPOs can be utilized for computing using coherent cluster states and Ising machines, in addition to their application as sources of broadband and quantum light for communications and sensing.
Reference
McKenna, T. P., Stokowski, H. S., Ansari, V., Mishra, J., Jankowski, M., Sarabalis, C. J., ... & Safavi-Naeini, A. H. (2022). Ultra-low-power second-order nonlinear optics on a chip. Nature Communications. https://www.nature.com/articles/s41467-022-31134-5
Disclaimer: The views expressed here are those of the author expressed in their private capacity and do not necessarily represent the views of AZoM.com Limited T/A AZoNetwork the owner and operator of this website. This disclaimer forms part of the Terms and conditions of use of this website.