Nd:YAG infrared laser as a viable alternative to excimer laser: YBCO case study
Sandeep Kumar Chaluvadi, Shyni Punathum Chalil,
Scientific Reports volume 13, Article number: 3882 (2023) Cite this article
Abstract
We report on the growth and characterization of epitaxial YBa\(_{2}\)Cu\(_{3}\)O\(_{7-\delta }\) (YBCO) complex oxide thin films and related heterostructures exclusively by Pulsed Laser Deposition (PLD) and using first harmonic Nd:Y\(_{3}\)Al\(_{5}\)O\(_{12}\) (Nd:YAG) pulsed laser source (\(\lambda\) = 1064 nm). High-quality epitaxial YBCO thin film heterostructures display superconducting properties with transition temperature \(\sim\) 80 K. Compared with the excimer lasers, when using Nd:YAG lasers, the optimal growth conditions are achieved at a large target-to-substrate distance d. These results clearly demonstrate the potential use of the first harmonic Nd:YAG laser source as an alternative to the excimer lasers for the PLD thin film community. Its compactness as well as the absence of any safety issues related to poisonous gas represent a major breakthrough in the deposition of complex multi-element compounds in form of thin films.
Introduction
Oxide perovskite thin films host innumerable properties in electronics, magnetism, and optics just by tuning/doping the cation elements as well as oxygen content1,2,3,4,5,6. Pulsed laser deposition (PLD) has become a state-of-art thin film growth facility in the oxide community after the successful demonstration of the stoichiometric transfer of superconducting YBa\(_{2}\)Cu\(_{3}\)O\(_{7-\delta }\) (YBCO) complex oxide7 by KrF excimer laser of wavelength \(\lambda\) = 248 nm. Since then, KrF excimer lasers have emerged as a dominant tool for the growth of very high-quality complex oxide thin films8,9,10,11 with applications ranging from fundamental material research to advanced semiconductor manufacturing industries for devices12,13,14. However, severe limitations arise regarding the use of excimer lasers in PLD laboratories worldwide. Excimer lasers consist largely of a mixture of noble gases (96% Ne, 3.5% of Kr/Ar) and the remaining 0.05% belongs to the halogen (i.e. F/Cl) mixture in He, present in the discharge chamber. The use of excimer lasers often raises concerns about safety issues (e.g. presence of highly poisonous gases) thus requiring expensive infrastructures to allow their use. Moreover, due to the ever-increase in demand and the scarcity of noble gas resources, the cost of KrF premix gas mixture has risen tremendously in recent past years. In this respect, to reduce their consumption, industries have also incorporated ways to recycle these gases and achieved up to 85% of the gas recycling ratio with stable laser energy output15. Yet, the wait time for the premix gas mixtures has severely increased in these last years thus not only hindering the smooth flow of the day-to-day activities but also tremendously increasing the maintenance costs of the lasers.
To lower the costs and long wait times incurred from the non-availability of noble gas mixtures for the excimer lasers, material scientists have started to incorporate solid-state Nd:Y\(_{3}\)Al\(_{5}\)O\(_{12}\) (Nd:YAG) lasers in the PLD growth process. Nd:YAG laser uses inorganic crystals for the production of highly energetic laser radiation, therefore, ruling out any safety issues regarding the presence of poisonous gases. The fundamental frequency of the Nd:YAG laser is at 1064 nm in the infrared (IR) region of the light spectrum, but by introducing the optical harmonic crystal generators, the wavelength of the laser can be pushed to the ultraviolet (UV) region i.e., 266 nm (4th harmonics) and 213 nm (5th harmonics) mimicking the excimer’s laser wavelengths. Though the use of higher harmonic generators has allowed the successful growth of oxide thin films16,17,18, limitations such as an enormous reduction in laser output energy by using harmonic generators which are known to possibly result in an incongruent ablation of the target19 and inhomogeneity in the laser beam profile have made them less attractive with respect to the excimer lasers.
By taking the advantage of the recent technological advancements in the Nd:YAG lasers such as uniformity in the overall laser beam profile20 and high stability in laser energy, we were able to deposit high-quality stoichiometric complex oxide thin films using only its fundamental harmonics at 1064 nm. In particular, the significant improvement in Nd:YAG laser technology has made it possible to produce extremely smooth films and avoid/minimize the number of droplets on the film surface. In our prior works, we have successfully demonstrated the utilization of fundamental harmonics (1064 nm) in the growth of epitaxial simple oxides such as TiO\(_{2}\)20 and V\(_{2}\)O\(_{3}\)21,22,23,24. Scanning tunnel microscopy (STM) and angular-resolved photo-emission spectroscopy (ARPES) experiments have indicated the excellent thin film surface quality created by Nd:YAG laser, when compared to the same materials grown by KrF excimer laser25,26,27,28. In this paper, we present yet another potential application of Nd:YAG laser operating at its fundamental harmonics namely, the stoichiometric transfer of complex oxide perovskite systems such as YBCO and LaNiO\(_{3}\) (LNO). The use of this kind of laser in the growth of very high-quality complex oxides represents a breakthrough in the project and realization of PLD facilities with very low maintenance costs and negligible safety issues regarding the presence of poisonous gases.
Experimental set-up using an Nd:YAG laser
The schematic of our PLD system using an Nd:YAG laser source is shown in Fig. 1. Differently from the KrF excimer laser characterized by a very large and heavy cabinet (e.g. 1182 \(\times\) 375 \(\times\) 793 mm and 275 kg of weight), the laser head of an Nd:YAG laser can be placed just in front of the entry-window. Such a possibility is allowed because of the sizeably smaller dimensions/weight of a typical Nd:YAG laser head. For instance, the Innolas SpitLight Compact 400’s laser head in use in our laboratory is 390 \(\times\) 135 \(\times\) 91 mm ((L \(\times\) W \(\times\) H) in dimensions for a total weight of about 10 kg.
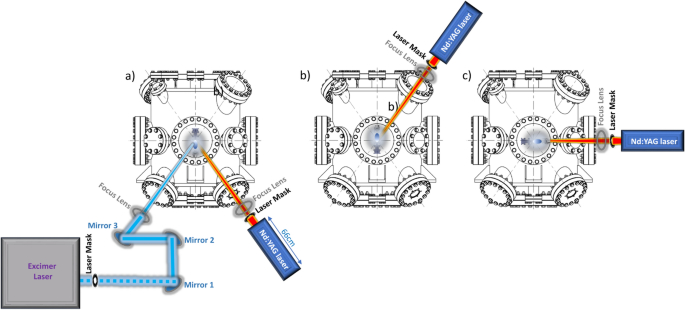
Schematic representation of our PLD system equipped with the Nd:YAG laser, laser mask, and focus lens (a) (typical laser-path of KrF excimer laser is also shown; other possible geometrical configurations of the laser head positioning (b and c).
Its positioning does not, therefore, require the use of reflecting mirrors to guide the laser pulses inside the deposition chamber thus allowing any possible geometry such as from the top or in the planar ones shown in a, b and c of Fig. 1, respectively. Moreover, the use of the full output energy of the laser is then allowed. The laser output energy is fixed at about 700 mJ with a beam diameter of about 6 mm and a maximum repetition rate of 10 Hz. Smaller repetition rates can be easily obtained by regulating the opening of the optical cavity and the actual number of laser shots per given time. It is crucial to underline the extremely high stability in the energy of the laser pulse (i.e. <0.7% ) making the ablation rate highly stable over time as well.
In contrast to KrF excimer lasers, where the laser output energy is controllable, the lack of Nd:YAG laser’s capacity to tune the energy has been circumvented by adopting a specific process protocol for the growth/optimization of epitaxial thin films. As a matter of fact, to reduce the total fluence of the laser pulse, an alumina ceramic piece with a variable diameter hole (e.g. 1−2 mm or larger/smaller) is used as a laser mask. After that, a focus lens has been placed in the laser path in order to focus the laser pulses onto the targets at an incident angle of 45∘.
Deposition of superconducting YBa2Cu3O7−δ single-layer thin films
YBCO belongs to the cuprates family and is well-known for its high superconducting critical temperature Tc at about ∼ 90 K. Bulk YBCO has an orthorhombic crystal structure (a = 0.384 nm, b = 0.393 nm, c = 1.182 nm, α=β=γ=90∘) and the space group belongs to Pmmm29. However, the growth of high-quality epitaxial YBCO thin films is the utmost priority for enabling their superconducting properties. Several works have been already done on the growth of very high-quality epitaxial thin films of YBCO on various substrates by PLD technique and exclusively by using KrF excimer laser. As an alternative to the use of excimer lasers, very few groups have also tried the deposition of epitaxial YBCO thin films on SrTiO3 or MgO substrates by using Nd:YAG laser either with 3rd (λ=355 nm)30 or 4th (λ=266 nm)16,31,32 harmonics as a pulsed laser source, respectively, both falling in the UV-range of the radiation spectrum.
In contrast to the preceding investigations, here, we yet again used the Nd:YAG laser as a pulsed laser source operating in its fundamental harmonics (i.e., λ=1064 nm) to demonstrate the growth of epitaxial YBCO films grown on LaAlO3 (LAO) [0 0 1] substrate. The crystallographic properties of YBCO films were checked by x-ray diffraction (XRD). In Fig. 2, the typical symmetrical θ−2θ scan shows only the YBCO (0 0 l) peaks denoted as ⧫, indicating highly textured growth along the c-axis direction. Other than the LAO substrate peaks (denoted by ◊), no further traces of impurity peaks or secondary phases can be observed.
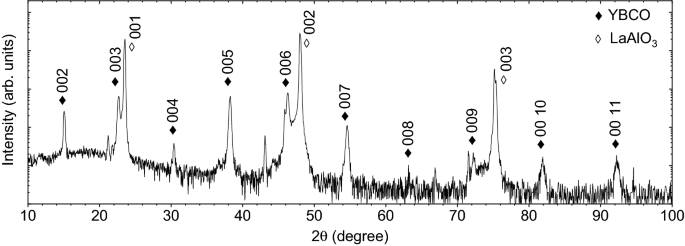
θ−2θ XRD scan of YBCO film grown on LAO [0 0 1] substrate.
The out-of-plane lattice parameter calculated from the (0 0 2) Bragg-reflection is ∼ 1.176 nm, very close to its bulk lattice constant29. In order to evaluate the effect of post-deposition thermal treatment, the transport properties of as-grown as well as different post-annealing conditions of YBCO films were investigated by the four-probe method.
The as-grown samples displayed very low Tc∼ 8 K. This feature can be explained by the low oxygen deposition pressure, i.e. 5 ×10−2 mbar, thus resulting in oxygen deficiencies in the YBCO as-grown films. To compensate for the oxygen deficiencies, the films were post-annealed at different annealing temperatures and oxygen pressure conditions. The films that were mildly post-annealed (500 ∘C, 100 mbar oxygen, 60 min) show an improvement in Tc from 8 to 62 K. Whereas, the film that underwent extreme post-annealing (600 ∘C, 300 mbar oxygen, 60 − 80 min) showed typical superconducting behavior with a linear slope, and its Tonsetc increased from 55 to 93 K, indicating an increase in oxygenation in the film.
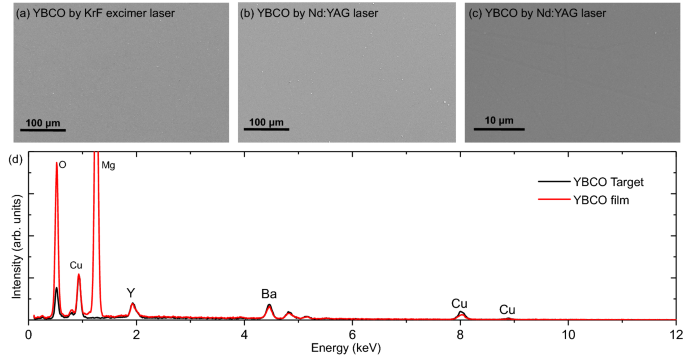
SEM images of the YBCO thin films grown using (a) KrF Excimer laser and (b and c) first harmonic Nd: YAG solid-state laser are presented. (d) Comparison of the EDS spectra of a YBCO target (black) and a YBCO film (red) grown by the first harmonic Nd: YAG laser.
To assess the microstructural surface quality of the thin films produced with the first harmonic Nd:YAG laser, a comparison of Scanning Electron Microscopy (SEM) images between the films grown using the conventional KrF Excimer laser and the new-generation Nd:YAG solid-state laser is presented in Fig. 3. Contrary to prior reports of droplets appearing in PLD-grown YBCO films33,34,35, the surface quality of the YBCO film grown with the first harmonic Nd:YAG laser, as shown in Fig. 3b and c, is smooth and free of droplets, similar to the film surface grown using the KrF laser (as depicted in Fig. 3a). The stoichiometric transfer of elements can be verified by comparing the Energy Dispersive Spectroscopy (EDS) line profiles of the YBCO target and the YBCO film, which are identical within the constraints of the instrumentation, as demonstrated in Fig. 3d. The structural, superconducting properties, microstructural and composition analysis of YBCO films reveal that the new-generation Nd:YAG lasers are equally comparable to the traditional KrF laser source.